MIT新型紧凑型聚变反应堆十年内实现发电
2015-08-14 10:41:56 来源:冷聚变世界翻译整理 评论:0 点击:
我们经常听说,30年就可以实现可控聚变技术发电,这个在科学界已经成为一个笑话了,今年说三十年,十年后还是三十年。如果按ITER的目前发展速度,我们很难在2050年大规模的采用聚变技术发电。MIT对于聚变发电提出了新的方案。
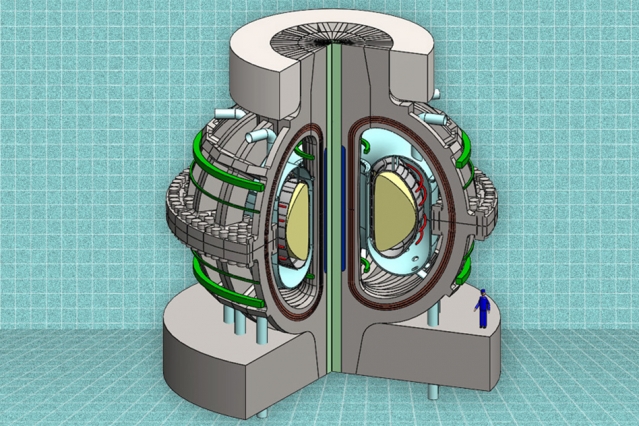
上图为:MIT提出的聚变反应堆外形
科学家们开始对听到聚变能源30年内就可以变为现实已经开始厌倦了,这已经是一个笑话了,永远都是三十年。
现在,这个笑话不再是真的了,先进的磁场技术使得MIT的研究人员提出了一种实用紧凑的托卡马克聚变反应堆的新设计,这种设计可以在10年内得以实现。获得取之不尽用之不竭聚变能源的世纪离我们越来越近了。
使用新型的商业化的稀土钡铜氧化物超导体可以制造出超强磁场线圈,这些线圈对于整个设计来是一种突破。MIT核科学工程系教授同时也是等离子体科学和聚变中心主任的Dennis Whyte说:它将会改变所有这一切。
强磁场可以制造出将超热等离子体约束所需要的条件,这些等离子体就是聚变反应的材料,这个装置比我们以前所设想的要小的多。体积缩小使得整个系统更加便宜,建设速度也更快,也使得一些天才的设计能够体现到电厂的设计上。设想的反应堆使用托卡马克形状(甜甜圈外形)已经被充分研究了,研究成果发表在聚变工程和设计期刊上,由Whyte,博士研究生Brandon Sorbom以及其他11位来自MIT人员。这篇论文开始于Brandon Sorbom所教授得课程最后在课程结束时转变成学生牵头的项目。
文章比较长,笔者没有翻译完,文章的主题意思是MIT的实验团队使用了一种更加强的磁场技术,可以将聚变反应器的尺寸缩小一半,而且能够实现发电。
冷聚变世界点评:就像文章的开头所说的,30年就可以实现可控聚变技术发电,这个在科学界已经成为一个笑话了,今年说三十年,十年后还是三十年。也不知道MIT的新反应堆设计能否获得国家资助,即使是获得资助,是否能获得成功。不过有一点可以确定的是,罗西及其工业热力公司已经开始冷核聚变技术的商业化了,等到可控热核聚变获得突破,冷核聚变技术早以其更小的体积,更高的效率,应用更广泛的特性占领市场了。
冷聚变世界
2015年8月14日
2015年8月14日
附文章地址和原文:
https://newsoffice.mit.edu/2015/small-modular-efficient-fusion-plant-0810
A small, modular, efficient fusion planthttps://newsoffice.mit.edu/2015/small-modular-efficient-fusion-plant-0810
New design could finally help to bring the long-sought power source closer to reality.
It’s an old joke that many fusion scientists have grown tired of hearing: Practical nuclear fusion power plants are just 30 years away — and always will be.
But now, finally, the joke may no longer be true: Advances in magnet technology have enabled researchers at MIT to propose a new design for a practical compact tokamak fusion reactor — and it’s one that might be realized in as little as a decade, they say. The era of practical fusion power, which could offer a nearly inexhaustible energy resource, may be coming near.
Using these new commercially available superconductors, rare-earth barium copper oxide (REBCO) superconducting tapes, to produce high-magnetic field coils “just ripples through the whole design,” says Dennis Whyte, a professor of Nuclear Science and Engineering and director of MIT’s Plasma Science and Fusion Center. “It changes the whole thing.”
The stronger magnetic field makes it possible to produce the required magnetic confinement of the superhot plasma — that is, the working material of a fusion reaction — but in a much smaller device than those previously envisioned. The reduction in size, in turn, makes the whole system less expensive and faster to build, and also allows for some ingenious new features in the power plant design. The proposed reactor, using a tokamak (donut-shaped) geometry that is widely studied, is described in a paper in the journal Fusion Engineering and Design, co-authored by Whyte, PhD candidate Brandon Sorbom, and 11 others at MIT. The paper started as a design class taught by Brandon Sorbom and became a student-led project after the class ended.
Power plant prototype
The new reactor is designed for basic research on fusion and also as a potential prototype power plant that could produce significant power. The basic reactor concept and its associated elements are based on well-tested and proven principles developed over decades of research at MIT and around the world, the team says.
“The much higher magnetic field,” Sorbom says, “allows you to achieve much higher performance.”
Fusion, the nuclear reaction that powers the sun, involves fusing pairs of hydrogen atoms together to form helium, accompanied by enormous releases of energy. The hard part has been confining the superhot plasma — a form of electrically charged gas — while heating it to temperatures hotter than the cores of stars. This is where the magnetic fields are so important—they effectively trap the heat and particles in the hot center of the device.
While most characteristics of a system tend to vary in proportion to changes in dimensions, the effect of changes in the magnetic field on fusion reactions is much more extreme: The achievable fusion power increases according to the fourth power of the increase in the magnetic field. Thus, doubling the field would produce a 16-fold increase in the fusion power. “Any increase in the magnetic field gives you a huge win,” Sorbom says.
Tenfold boost in power
While the new superconductors do not produce quite a doubling of the field strength, they are strong enough to increase fusion power by about a factor of 10 compared to standard superconducting technology, Sorbom says. This dramatic improvement leads to a cascade of potential improvements in reactor design.
The world’s most powerful planned fusion reactor, a huge device called ITER that is under construction in France, is expected to cost around $40 billion. Sorbom and the MIT team estimate that the new design, about half the diameter of ITER (which was designed before the new superconductors became available), would produce about the same power at a fraction of the cost and in a shorter construction time.
But despite the difference in size and magnetic field strength, the proposed reactor, called ARC, is based on “exactly the same physics” as ITER, Whyte says. “We’re not extrapolating to some brand-new regime,” he adds.
Another key advance in the new design is a method for removing the the fusion power core from the donut-shaped reactor without having to dismantle the entire device. That makes it especially well-suited for research aimed at further improving the system by using different materials or designs to fine-tune the performance.
In addition, as with ITER, the new superconducting magnets would enable the reactor to operate in a sustained way, producing a steady power output, unlike today’s experimental reactors that can only operate for a few seconds at a time without overheating of copper coils.
Liquid protection
Another key advantage is that most of the solid blanket materials used to surround the fusion chamber in such reactors are replaced by a liquid material that can easily be circulated and replaced, eliminating the need for costly replacement procedures as the materials degrade over time.
“It’s an extremely harsh environment for [solid] materials,” Whyte says, so replacing those materials with a liquid could be a major advantage.
Right now, as designed, the reactor should be capable of producing about three times as much electricity as is needed to keep it running, but the design could probably be improved to increase that proportion to about five or six times, Sorbom says. So far, no fusion reactor has produced as much energy as it consumes, so this kind of net energy production would be a major breakthrough in fusion technology, the team says.
The design could produce a reactor that would provide electricity to about 100,000 people, they say. Devices of a similar complexity and size have been built within about five years, they say.
“Fusion energy is certain to be the most important source of electricity on earth in the 22nd century, but we need it much sooner than that to avoid catastrophic global warming,” says David Kingham, CEO of Tokamak Energy Ltd. in the UK, who was not connected with this research. “This paper shows a good way to make quicker progress,” he says.
The MIT research, Kingham says, “shows that going to higher magnetic fields, an MIT speciality, can lead to much smaller (and hence cheaper and quicker-to-build) devices.” The work is of “exceptional quality,” he says; “the next step … would be to refine the design and work out more of the engineering details, but already the work should be catching the attention of policy makers, philanthropists and private investors.”
The research was supported by the U.S. Department of Energy and the National Science Foundation.
分享到:
收藏

评论排行
- ·黑光能源公司 BlackLight Power, In...(4294967281)
- ·冷聚变技术可以结束当前的雾霾天气吗?(13)
- ·布里渊能源技术公司的CECR技术简介(9)
- ·Defkalion公司的5千瓦Hyperion冷聚变反应堆(8)
- ·截止2014年国内外冷聚变研究现状(8)
- ·美军宣称已成功将海水直接转化为燃料(8)
- ·关于近期对E-CAT真实性质疑的问题(8)
- ·中核研究院成功复制镍氢冷核聚变装置E-CAT(7)
- ·美预言家预测人类在未来18个月内实现冷...(6)
- ·黑光能源公司宣布改变能源领域游戏规则...(6)
- ·镍氢电能(E-CAT)研究中心落户天津(6)
- ·工业热力公司公告称罗西起诉没有任何价值(6)
- ·空中客车公司对冷核聚变研发感兴趣(5)
- ·1升水能让汽车跑5000公里(4)
- ·冷聚变开拓者:马丁. 弗莱希曼 和 斯...(4)
- ·世界上第一台冷聚变装置E-CAT的研发历程(4)
- ·冷聚变是伪科学吗?(4)
- ·冷聚变将引发环保和新能源技术新的革命(4)
- ·德国EGM公司的水基燃料技术(4)
- ·英国国防部最新报告:冷聚变技术将来会...(4)